FlyONSPEED.org Reduce Loss of Control Risk

Winner 2018 EAA Founder's Innovation Award and 2021 Grand Champion Winner
Energy Management 101
Using the AOA Tone to Assist with Energy Management
What is "Energy" and how do we manage it?
Energy is power converted into airspeed and altitude. It’s intuitively obvious that if we push the throttle up, we can go up or go faster—until we run out of “energy,” then we can’t. Energy can be “negative” and no matter what we do with the throttle, we may not be able to accelerate or climb. For a simple mental model, we can pare down the concept even more: Energy = altitude + airspeed. In powered airplanes, we control energy with pitch, power and roll inputs. How we control energy during flight is called “energy management” (EM). EM is how we manage airspeed and altitude using flight and power controls. We use power to add energy, and pitch to swap airspeed and altitude (by controlling the amount of lift and drag). The pitch reference AOA we care about during maneuvering is ONSPEED. It is an important line of demarcation. At ONSPEED AOA, thrust and drag are balanced. Slower than ONSPEED, there is more drag than thrust; faster, there is more thrust than drag. In addition to ensuring we have sufficient energy; we want to avoid stalling unintentionally. In general, we want to maintain ONSPEED or faster, since energy is negative and aerodynamic margin is reduced if we fly slower than ONSPEED.
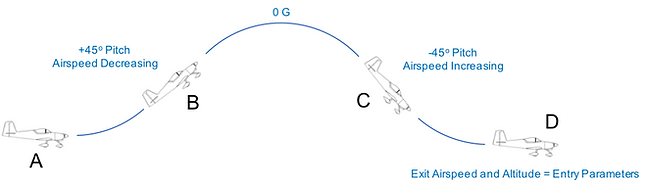
Pitch
Figure 1 is an easy way to visualize energy during a maneuver. At position A, the airplane is at cruise speed and the pilot smoothly pulls the nose up until his heels are on the horizon (about 30-45o nose up) without adjusting power. As the nose comes up, airspeed decreases, and altitude increases. At Point B, the pilot smoothly eases the stick forward to establish a low-G (<1) condition. As long as the pilot maintains a low-G condition using pitch (AOA), the airplane will fly a ballistic arc over the top of the maneuver. At zero G, stall speed is zero, so IAS may be quite low at the apex. In the first half of the maneuver, the pilot has simply traded kinetic energy (airspeed) for potential energy (altitude) using pitch. If the pilot continues to fly to 30-45o nose down, airspeed will begin to increase, and altitude will decrease. A smooth pull back to level flight will establish the same altitude and airspeed at point D that the airplane had at Point A. Physics majors will note that energy throughout this maneuver remains constant. Pilots will note that if we pull the nose up X degrees, we’ll have to push it down X degrees to get back to where we started as efficiently as possible.
Power
We learned about the power required curve and the “region of reverse command” during training. Let’s condense these concepts into a simple model: from the perspective of throttle, if we are faster than minimum power required AOA/speed, there is excess power available, and we can accelerate or climb if we push the throttle up. If we are slower, even if we push the power up, we can’t (Figure 2).
Figure 2

Roll
When we roll, we are adjusting where lift is pointed. In level flight, all lift is going up and in inverted flight, all lift is going down. Banked < 90 degrees, some lift is going up, and banked > 90 degrees, most lift is going down. This is why airplanes accelerate so fast inverted, nose low: thrust, lift AND weight all contribute to the thrust vector. An easy way to picture this is a loop: a purely vertical 360-degree turn. In a loop, all of the lift the airplane is producing is used to turn. In a level, upright turn some of the lift turns the airplane, and some of the lift counters weight. Figure 3 depicts the “horizontal component of lift” we learned about in training. In this example the airplane is banked 45o, so it’s necessary to pull 1.4 G’s to maintain level flight. Because 1.4 G’s requires extra lift, extra drag is generated, which is why you have to push the power up in a steep turn to maintain a constant airspeed. More energy is required; and in this case, you are getting it by adding power. Another way to sustain energy is to trade altitude for airspeed, so if you didn’t throttle up for the steep turn, you could let the airplane descend during the turn to maintain speed. This simple algebra of swapping airspeed and altitude, and using the throttle is what energy management is all about.
Figure 3

Figure 4 depicts a simple aileron roll. If the pilot starts to roll the airplane without pulling up at the start of the roll, the airplane will be nose down at the end. That’s because the net amount of lift in an upward direction is a wash: half of the maneuver it’s up, and half it’s down. The airplane behaves like a ball thrown straight ahead. The amount of drop depends on how fast the airplane rolls—faster roll rate means less drop and vice versa. Because the airplane is accelerating downhill, total energy at the end of the maneuver equals total energy at the start of the maneuver.

In Figure 5, the pilot pulls the nose up to manage energy using the “angles = angles” technique. In this example the horizontal trajectory of the airplane is an arc like Figure 1 and degrees nose down in the second half of the roll equal degrees nose high in the first half—allowing a smooth recovery to wing’s level at entry altitude at entry airspeed and altitude.

Figure 5
The Basics
EM is best conceptualized as a problem with three variables: speed, altitude and power. If only one variable is changing, then it’s pretty easy to figure out how to manage energy. At its most basic, we can generally think of EM in terms of controlling speed: how we go fast or slow or how we stabilize speed (or AOA) where we want it. If we are fast, we’ll either reduce power, pitch up (increase AOA and G) or apply some of each correction. If we are slow, we either add power, pitch down (reduce AOA and G) or some combination of both. If we modify Figure 2 slightly, we come up with the “push/pull” model depicted in Figure 6. It is important to note is that if you are slower than ONSPEED and at full throttle, then the only way to accelerate is to “unload” (reduce pitch/G/AOA) and trade altitude for airspeed. This is why at low altitude, just avoiding a stall isn’t enough—you also have to maintain sufficient energy to avoid hitting the ground.
Figure 6

Turning
Why is ONSPEED a Goldilocks condition, where everything is “just right” in terms of turn performance? In technical terms, it means Ps = 0, where Ps refers to aircraft specific energy: a blend of speed, thrust, drag and weight. Maximum sustained turn rate and minimum sustained turn radius occur ONSPEED. This is referred to as “optimum turn.” In simple terms: it’s the best you can do with the energy you have. If you pull any harder than ONSPEED, you are venturing into the left side of Figure 6 and no matter what you do, you will slow down, go down, or both. Another way to picture this is to depict the ONSPEED band (ONSPEED AOA ± approximately 1 degree) on the flight envelope (Figure 7).

Figure 7
In Figure 7, the portion of the flight envelope colored yellow is same as the left side of the vertical line in Figure 6. If you are in this area, the airplane is going to slow down, even if you push the power all the way up. You can avoid this deceleration somewhat by trading altitude for airspeed (letting the airplane descend), but the supply of altitude is always finite. Let’s look at a special case: maneuvering speed or “corner velocity.” This is the point in the upper left-hand corner of the envelope where the airplane can pull maximum allowable G at minimum airspeed and achieve maximum instantaneous turn performance. The key word in the last sentence is “instantaneous.” There is no way to sustain this condition in a level turn. If a pilot flying the airplane depicted in Figure 7 was in level flight at maximum forward speed, banked, maintained full power and pulled smoothly to the 6 G limit, the airplane is going to slow down. All those G’s create lots of drag due to lift, and that drag will slow the airplane down quickly. If the pilot maintains 6 G’s nearly perfectly, the airplane will stall at maneuvering speed at 6 G’s and about 135 KTS/MPH IAS. If, however, the pilot initially pulled 6 G’s, then relaxed back pressure at ONSPEED AOA, and maintained ONSPEED, turn rate and radius would stabilize at maximum sustained turn performance (i.e., optimum turn) and no stall will occur. Thus, the dark green band in Figure 7 represents the combination of airspeed and G that balance thrust with drag. This does not, however, mean the airplane can sustain level flight at G loads greater than 1. That ability is purely a function of power available.
P Sub S
P sub S. When we think in terms of energy and energy management, it becomes obvious that avoiding loss of control means more than just avoiding a stall and spin. Controlling AOA (and sideslip) keeps you from stalling and spinning; but managing energy keeps you from running out of altitude. Specific Excess Power (abbreviated as Ps and pronounced “P sub S”) measures energy rate of change—the airplane’s ability to climb or accelerate from a given flight condition. “Given flight condition” is a snapshot of current speed, density altitude, weight, g-load and propeller thrust. When the value of Ps is positive the airplane can climb and/or accelerate. When the value of Ps is negative the airplane will NOT be able to climb or accelerate. Here is how Ps is calculated:
Ps = V(T-D/W)
Where D = drag, V = velocity, T = thrust and W = weight. We can see that when drag exceeds thrust for a given weight, energy is negative. Weight is a function of G load. As G increases, more thrust is required to overcome drag. We can get that thrust by pushing up the power lever and/or by converting altitude into thrust by descending.
Since AOA is independent of density altitude, weight and G-load, we can simplify energy management in everyday flying by applying the AOA tone logic. Under positive G, no tone, or fast tone means that overall energy is positive, ONSPEED (steady tone) indicates energy is neutral and slow tone indicates energy is negative. While PS is independent of stall, the nifty thing about the tone logic is that it not only provides an energy cue under positive G, but the slow tone also provides an indication of aerodynamic margin—i.e., how close to the stall we are. With flaps up, when we are ONSPEED we have maximum thrust available and when we are at the start of the fast tone (L/Dmax) we have maximum power available.
The faster you go, the faster you go faster. This is because power is thrust x velocity: if you are faster, you simply have more power available. A hypothetical airplane at gross weight, full throttle and level at 10,000 feet and 70 knots may be above stall but too slow to accelerate without first descending. But the same airplane at 90 knots at 10,000 feet may have positive Ps and be able to accelerate.
Turn Performance
When we maneuver close to the ground (during takeoff and landing) we are really concerned with turning room: do we have enough room to turn without hitting the ground, or anything attached to the ground? Or, if the engine fails, how far can with turn before we hit the ground, or anything attached to the ground? When an airplane turns, maximum instantaneous turn performance (i.e., smallest turn radius and maximum turn rate) occurs at a maximum lift. But we know intuitively that there isn’t sufficient power to sustain this condition if the thrust to weight ratio of the airplane is less than 1:1. Maximum sustained turn performance (i.e., best turn rate that can be sustained over time with energy available) occurs at 0 Ps: when thrust and drag are balanced.
Figure 8 shows PS vs turn rate. Note that maximum sustained turn occurs ONSPEED and maximum instantaneous turn occurs when the airplane generates maximum lift. Turn performance decreases as the airplane approaches the aerodynamic limit (the yellow area in Figure 7 above). Also note that any time overall energy is negative under positive G, slow tone is present.

Figure 8
Another way to depict turn performance is a rate/radius diagram. A diagram for a typical EAB airplane is shown in Figure 9. This diagram shows turn rate and radius vs speed. The solid black line is maximum instantaneous performance, and the apex occurs at corner velocity/maneuvering speed. The solid red line depicts maximum sustained turn performance. This occurs ONSPEED.
The important lesson to glean from the turn and Ps discussion and Figures 7-9 is that for normal flying, don’t pull harder than ONSPEED when you maneuver. If you do, you are expending energy for no net gain in performance and are operating with reduced aerodynamic margin.

Figure 9
Climbing
One way to think about energy during takeoff and climb is how efficiently are we converting power into altitude? Obviously, the variable is airspeed, but again, we can substitute ONSPEED and L/Dmax AOA for airspeed. If we climb ONSPEED, the airplane will climb as rapidly as possible for a given distance (best angle) and if we climb at L/Dmax the airplane will climb as rapidly as possible over distance (best angle) and if we climb at L/DMAX the airplane will climb rapidly over time (approximating best rate). The “don’t pull harder than ONSPEED” rule applies during climb as well, because climbing slower than ONSPEED, results in decreased climb performance (you are trying to fly in the yellow region in Figure 7).
Approach and Landing
Landing speed is another Goldilocks parameter: it has to be “just right,” not too fast and not too slow. Because IAS is affected by gross weight, load factor (G/bank angle) and density altitude; it’s generally easier to reference AOA for approach and landing because ONSPEED AOA is always the same (it’s designed into the airplane). Figure 8 is an energy efficient visual landing pattern. It’s energy efficient, because it allows the airplane to achieve stable parameters prior to the final alignment phase of landing without the use of power, if desired. It should look similar to the “power-off 180-degree landings” we practiced when we learned to fly. It’s designed to allow the pilot to concentrate purely on flying the airplane from the time the base turn is started through landing.
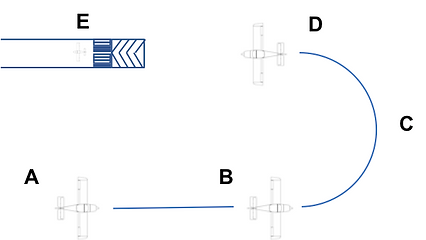
At Point A, the airplane should be no faster than L/Dmax and landing checks should be complete. If equipped, partial flaps may be set by this point. The objective is to arrive at Point B (the beginning of the base turn) ONSPEED in the landing configuration (i.e., landing flaps and gear, if applicable). From Point B to the runway over-run, the pilot maintains an ONSPEED condition using pitch control, based on feedback from the AOA tone. Because we are making a continuous turn from downwind to final, it’s easy to compensate for any cross-wind effect (over- or under-shooting) by varying the amount of bank we use to turn. The pilot should visually pick out a point on the ground on the extended runway centerline over which to roll-out for final and adjust bank during the base turn to roll-out over the top of that point. The aural AOA logic accommodates bank changes and provides precise pitch control feedback to the pilot. If equipped with a stereo intercom or headset, the logic also provides sideslip information by slewing the tone in the sound field to follow the slip/skid ball. Aural cues simplify visual cross-check and increase “eyes out” of the cockpit time.
Pattern size depends on the power setting used in the base turn and on final approach. IDLE power requires a tight pattern but is the most energy efficient way to get the airplane to a stable ONSPEED condition prior to touchdown.
As a technique, consider flying this power off pattern every time you land to maintain proficiency (traffic permitting). I take Bob Hoover’s advice to heart that I need to think of energy as money in the bank best taken out in small increments. In other words: several small corrections are better than one big one for us less than perfect aviators. You can always “bleed” a bit of energy with a slip if you’re already in IDLE or add a touch of throttle if you need to adjust glide path angle or change your ground track to the runway. If the airplane has more drag in the landing configuration (e.g., one with a controllable pitch propeller), it may be more comfortable to carry some power through the base turn. When you roll out on final approach, 10-12 seconds prior to touchdown, adjust pitch to maintain ONSPEED and power to stabilize glide path. After making final alignment corrections and transitioning to the flare (passing the over-run or crossing the fence), slow to a “slightly slow” tone for touchdown. If it’s gusty or turbulent, carry a bit of extra energy and fly a “slightly fast” final—the equivalent of flying Vapp vs Vref. Don’t get any slower than ONSPEED until you’re ready to land.
The aural AOA logic makes pitch control straight forward: if you want to be ONSPEED and hear any slow tone, ease some back pressure on the stick. This will happen anytime you are ONSPEED and increase bank angle. Add power during the correction if you need to control glidepath. If you hear any fast tone, then ease the stick back. If you heard the fast tone because you dropped the nose to maintain glidepath, then correct AOA with pitch and reduce power to keep the airplane descending on the desired glidepath. If you are already at IDLE, then some inside slip will help with the glide path if you are high. Several small corrections are always better than one big one.
Gliding
Once the engine stops on a single engine airplane, managing available energy is of paramount importance. Because power is no longer contributing to the energy equation, the only variables left are altitude and airspeed. There are two emergency glide parameters we learn about during training: maximum range glide and maximum endurance glide. Maximum range glide occurs flaps up at L/Dmax AOA and maximum endurance glide occurs at ONSPEED AOA. So, another simple EM rule of thumb is that if the engine quits, fly the airplane at L/Dmax or ONSPEED. As flaps are deployed, the speed for L/DMAX decreases the AOA changes, and the two cues begin to marry up: At full flaps, optimum glide performance occurs ONSPEED.
Basic EM Rules of Thumb
-
ONSPEED for optimum turn; approach and landing; best angle of climb and maximum endurance glide.
-
Don’t pull harder than ONSPEED unless you need maximum instantaneous aerodynamic performance (e.g., trying not to hit the ground or another airplane).
-
L/DMAX to approximate best rate of climb; for maximum range; and maximum range glide.
-
During approach and landing, do what it takes to maintain ONSPEED until committed to land.
-
It’s OK to think of AOA and energy in terms of “fast” or “slow.”
-
We add energy with throttle, we “spend” it with drag.
-
Several small corrections are better than one big one.
-
If the engine quits, fly L/Dmax or ONSPEED.